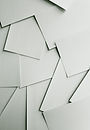
RESEARCH
The common theme that underlies all our research is self-assembling hybrid protein-DNA and peptide-DNA nanomaterials. We seek to merge the programmability of DNA nanotechnology through the functionality and structural diversity of proteins, which in turn requires site-specific biological conjugates. Four broad areas of interest include:
Structural protein-DNA nanotechnology
Biological systems like cells are a marvel of self-assembling protein nanostructures, which carry out functions like signaling, mechanical support, ligand binding, or intracellular transport. Despite the great chemical diversity of proteins, however, it is still challenging to rationally design nanostructures from scratch. DNA nanotechnology, by contrast, has the advantage of programmability thanks to the specificity of Watson-Crick pairing, but at the expense of chemical and functional diversity. We aim to merge protein and DNA nanotechnology, by integrating self-assembling protein motifs such as coiled-coils, oligomeric assemblies, or protein-protein interactions with DNA nanostructures. This in turn requires the use of multiple, site-specific bioconjugation reactions to attach oligonucleotide handles to the polypeptide molecule. We also explore non-covalent approaches for modifying proteins and peptides with DNA, including protein-based interactions (e.g. coiled-coils) or completely synthetic motifs (e.g. host-guest chemistry).

We aim to create both symmetric structures like fibers, sheets, or 3D cages, and highly anisotropic materials that approach the complexity of DNA origami. We envision these hybrid materials as nanoscaffolds for targeted cargo delivery (“artificial viruses”), development of antibody mimics, as well as the synthesis of vaccines or other immune-modulatory materials, and molecular machines/“nano-bots.”
Protein/peptide-DNA bio(nano)materials
In addition to their fascinating structural properties, proteins and peptides have a wealth of promising functional attributes for biology and medicine. This area of lab research involves making biomaterials (such as dynamic surfaces/hydrogels) and nanomaterials (e.g. synthetic antibodies, vaccines, drug delivery vehicles) using DNA as a functional linker, or as a scaffold/therapeutic cage. We also focus on coating DNA nanostructures with peptide and protein coatings, essentially functionalizing the DNA “skeleton” with a bioactive protein/peptide “skin.” The subset of projects in this area include:​
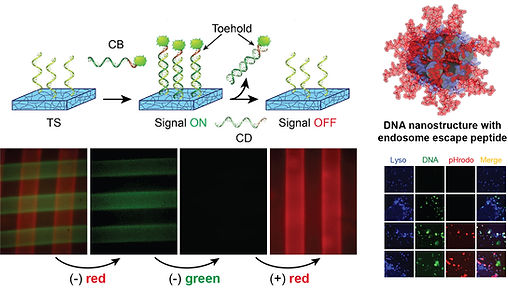
-
Tissue engineering biomaterials that use DNA as a dynamic trigger to reversibly control the stiffness and presentation of (multiple) bioactive presentation in both space and time
-
Synthetic antibodies that use DNA scaffolds to position binding peptides and proteins in 3D, for applications in targeting and therapeutics
-
Functionalizing DNA nanostructures with proteins and peptides in order to enhance targeting, uptake, endosomal escape, and cargo delivery inside of cells
-
Nanomaterials to modulate the immune response, especially through delivery of siRNA or mRNA into cells, as well as vaccine platforms
For all these projects, we collaborate extensively with biologists, engineers, and doctors to validate our materials in vitro, and eventually transition them to in vivo applications in tissue engineering and regenerative medicine.
DNA-templated synthesis of proteins and protein nanostructures
Oligonucleotides are a remarkable template for the sequence-specific synthesis of other molecules, with a prime example being mRNA translation into an amino acid polymer by the ribosome. We aim to use programmable DNA handles and scaffolds to synthesize novel materials driven by the co-localization and spatial control of the DNA to position peptides and proteins. We are investigating two key areas: (1) Synthesis of full-length proteins from individual peptide fragments via sequential, templated native chemical ligation; and (2) Synthesis of anisotropic protein nanostructures using DNA “assemblers” that position the individual components in space. Our ultimate goal is to synthesize full-length functional proteins “from scratch” (including ones that incorporate many synthetic residues) and “3D protein nano-printing” to build protein structures that approach DNA origami in complexity.

Self-assembled DNA crystals as macromolecular scaffolds
The foundational goal of DNA nanotechnology, as outlined by Ned Seeman in 1982, is to use self-assembling DNA strands to construct 3D crystals with defined cavities, in order to artificially “crystallize” other guest molecules like proteins. In collaboration with the lab of Prof. Hao Yan at ASU, we are exploring both the design principles for novel crystal lattices—with varying cavity sizes and symmetries—and the attachment of guest species like small molecules, catalysts, nanoparticles, peptides, and proteins in these crystals with atomic precision. These materials can be used for both structural elucidation of the guests, as well as to create novel materials from the 3D positioning of functional molecules like enzymes, catalysts, or drugs.
